Research article/ Open Access
DOI: 10.31488 /bjhd.113
Shear Wave Elastography as a Novel Ultrasound Method for Monitoring Exercise Capacity of Patients with Congestive Heart Failure
M. Maslarska, C. Bode, C. Hehrlein
1. Department of Cardiology and Angiology I, University Medical Center Freiburg, Hugstetterstr. 55, 79106 Freiburg, Germany
*Corresponding author: Mariya Maslarska, M.D, Department of Cardiology and Angiology I, University Medical Center Hugstetterstr. 55, 79106 Freiburg, Germany, Tel:+49/761 270 77090; Fax+49/761 270 32540.
Abstract
Aim: Ultrasound shear wave elastography (SWE) is a novel non-invasive method to detect exercise-induced peripheral muscle perfusion deficits in patients with chronic heart failure or clogged, incompressible arteries. We present the scientific background of muscle SWE and focus on its application for patients with chronic congestive heart failure with and without peripheral arterial disease. Methods and Results: This easy-to-perform, standardized technique of ultrasound uses maximal active muscle flexion or extension (M. flexor carpi, M.soleus) as an established surrogate parameter for exercise capacity of the extremities. SWE allows quantification of exercise related muscle function during different stages of chronic heart failure with a robust cuff value of 70 kpa. In this article, we discuss methods and pitfalls of peripheral muscle SWE in comparison with healthy subjects by reviewing of current literature. Conclusion: This novel ultrasound method helps to monitor improvements of muscular exercise capacity in heart failure patients during medical therapy and physical rehabilitation. Shear wave elastography may particularly be helpful in assessing therapeutic success when other diagnostic tools of muscular perfusion measurements such as magnetic resonance imaging (MRI) are cumbersome to perform.
Keywords: shear wave elastography, musculoskeletal ultrasound, congestive heart failure, peripheral arterial disease
Introduction
Shear wave elastography (SWE) is a novel ultrasound method, gaining popularity in the diagnosis of musculoskeletal disorders and lesions in parenchymal organs. Shear wave elastography applies high frequency shear waves, which propagate with a certain velocity depending on the tissue density [1,2]. In this way, the tissue`s elasticity presented by the shear modulus could be defined as the product of shear wave velocity and tissue density [3,1]. Images are visualised as a region of interest (ROI) within the examined system and superimposed on a B-Mode ultrasound image. A color-coded scale depicts the changes in the elasticity level. A transition from blue to red indicates an increase in the shear modulus or shear wave velocity, depending on the ultrasound machine [4,3]. Shear modulus is measured in kilopascal (kPa) and shear velocity in meters per second (m/s) [5,3]. The size and depth of the region of interest could be adjusted individually. Figure 1 demonstrates a SWE-image of the gastrocnemius muscle at rest with a color-coded scale (Figure1).
Shear wave elastography (SWE) found a major field of application in the evaluation of liver stiffness in chronic liver disease as well as focal liver lesions [6]. In their prospective study, Ferraioli et al. compared the diagnostic potential of point shear wave elastography (PSWE) and transient elastography (TE) in the assessment of liver fibrosis. They found a significant correlation between PSWE-measurements and the fibrosis stage in 102 patients with chronic hepatitis. Furthermore, healthy patients presented a median PSWE-value of 3,53 kPa compared to 5,34 kPa in the early fibrosis stages [7]. Moreover, shear wave elastography demonstrated very high diagnostic accuracy in patients with advanced fibrosis und cirrhosis [8].
Another field of interest in the use of shear wave elastography is the differentiation of malignant and benign breast lesions. In their prospective study of 119 women with a single breast mass Hari et al. obtained elasticity values for 57 benign and 62 malignant lesions. Benign breast masses were characterised by lower elasticity values with a mean of 47.9 kPa ±44 compared to 163kPa ±51.6 in the malignant group. Moreover, the authors describe a significant relationship between the visual colour measurement and the obtained elasticity value. In their results, they suggest a cut-off of 102 kPa in the quantitative discrimination of malignant and benign lesions. Chronic inflammation might present a diagnostic challenge since it can be depicted as both soft and stiff lesions [9]. In another original publication with a similar patient population Olgun et al. obtained stiffness values for malignant masses with different histological grades of malignancy. Low-grade or in situ tumours presented a mean elasticity of 64,3kPa as opposed to grade 2-3 lesions with a mean of 162,5kPa [10].
Recently there has been growing interest in the potential of shear wave elastography for the evaluation of diffuse thyroid disease. Patients with Hashimoto`s thyroiditis or Grave`s disease present significantly higher measurements of shear wave velocity compared to healthy subjects. However, a differentiation between both diseases solely based on the elasticity properties might be challenging since both groups are characterised by a certain degree of fibrosis and inflammation [7,11].

Figure 1.Shear wave elastography of the gastrocnemius muscle
(Original Image)
The region of interest is positioned in the middle of the coloured
square. The color-coded scale on the left side measures the shear
modulus in a range from 0kPa to 300kPa
In the last few years shear wave elastography has established its role in the diagnosis of musculoskeletal disorders and the quantitative evaluation of muscle function. In a prospective study of twenty-four patients with inflammatory myopathy (dermatomyositis and polymyositis) Botar-Jid et al. performed elastographic examinations of superior, inferior and medium muscle groups in the upper and lower extremities. Sonoelastography proves to be a useful diagnostic tool to detect dynamic changes in muscle structure and to quantify these alterations by measuring muscle stiffness. Furthermore, decreased muscular elasticity might be explained by the presence of fibrosis and atrophic changes similar to other organ systems [12].
Shear wave elastography provides quantitative information about muscle force as described in the original study of Leong et.al., where they examined the upper trapezius muscle at rest and during abduction at 30 degrees and acquired the shear elastic modulus for both states. An average elastic modulus of 17.1kPA±5,8 was measured during rest, whereas the elasticity increased up to 26,6kPA ±12,3 during an active muscle contraction [13]. In another experimental design the shear elastic modulus was compared to the electromyographic activity of the biceps brachii muscle. A significant correlation between these variables was detected corroborating the fact that muscle tension during a contraction is related to its elastic modulus [14].
The elastic properties of the lower muscle groups were examined in the study of Dubois et al, which included ten healthy participants without underlaying musculoskeletal disorders or traumas. The team performed measures at rest and during passive stretching of eleven muscles responsible for the knee joint`s activity and plantar flexion. Resting muscles of the lower extremity presented coherent elasticity measures with a range from 3,9 to 6,6 kPa, depending on location and muscle size. However, the shear modulus was significantly higher during passive stretching for all muscles except for the soleus muscle. This observation might be explained through the limited role of the soleus muscle in the knee joint motion [15].
Several original publications have evaluated the diagnostic reproducibility of shear wave elastography. The study of Leong demonstrated an intraclass correlation coefficient (ICC) of 0,78 at rest and 0,83 at a 30-degree obduction for the inter-operator variability of the upper trapezius muscles. ICCs between investigators range from 0,87 to 0,91 for the gastrocnemius and vastus medialis muscles at rest and during stretch [15]. In our original publication we provide similar results for the lateral gastrocnemius muscle from both sides [4]. Furthermore, certain technical aspects regarding the muscle structure should be considered in order to ensure the diagnostic repeatability. Shear wave elastography examinations should be performed with minimal transducer`s pressure to ensure reliable elasticity measures [16]. The transducer should be held in a longitudinal direction along the muscle fibres to provide consistent shear modulus values [17,18]. Methodological studies of the lower limb muscles have demonstrated that ROI`s size and the examined muscle`s side do not have any influence of the elasticity. Furthermore, it has been proposed that each muscle group presents an unique elastic modulus [19].
Technical Methods
Muscle contraction (exercise) demands increased blood perfusion in comparison to a resting state of the muscle. Central mechanisms such as an increased cardiac output and venous return as well as local interactions in the vascular resistance in activated muscles play an essential role in the regulation of peripheral muscle perfusion [20,21].
Delp and Laughlin postulate that resting skeletal muscles present low blood flow levels compared to other muscle groups due to a relatively high resistance of the local blood vessels combined with an increased sympathetic activity [22-25].
The vasomotor tone of humans allows a quick and sensitive correction in the capillary perfusion depending on the muscle activity and metabolic demands [22].
We previously showed in a clinical pilot study that ultrasound SWE reliably measures an impaired muscle stiffness during exercise as surrogate parameter of perfusion deficits in chronic heart failure patients. However, only exercise but not resting muscle stiffness values are of use. Control patients and heart failure patients presented similar resting results with a median SWE of 13.1 kPa for the controls and t12.5kPa for the heart failure group [4]. Thus, we conclude that SWE might only provide reliable information about a limited muscle perfusion during a maximal muscle effort.
In physiological terms muscle perfusion is directly dependant on the exercise activity [20,21]. A central mechanism is an increased cardiac output during exercise combined with a higher venous return to the heart. The later one is generated through muscle contractions known as the muscle pump, which compress venous segments and thus direct the blood flow towards the heart [22].
By referring to this mechanism, we proposed that chronic heart failure (CHF) patients presenting with an impaired peripheral exercises capacity can be diagnosed by their performance during a stretching exercise as surrogate parameter of muscle contraction [4]. Thus, we designed our study by excluding local muscle damage, muscle reactions such as metabolically induced vasodilatation and inflow obstructions such as arterial or venous stenosis in this assessment of muscle perfusion by SWE during muscle contraction [22].
In our original publication we presented healthy participants and heart failure patients, performing controlled muscle exercises, defined by an active maximal foot dorsal extension in a prone position or maximal flexion of underarm muscles via a handgrip manoeuvre. Dorsal foot stretch was performed via an active pull movement towards the frontal leg side for two minutes at maximum active force [4]. By these means, we observed sufficient tension in the lateral gastrocnemius muscle during ultrasound imaging. We identified a region of interest (ROI) within the gastrocnemius muscle during stretch, depicting the contraction level with shear wave elastography. Figure 2 shows the peripheral muscle of a healthy participant without any other morbidity during active muscle stretch. Figure 3 depicts an ultrasound examination of a heart failure patient without any other co-morbidity performing the same exercise. (Figure 2 and 3) Patients with peripheral arterial disease as a confounder were excluded from the study cohort.

Figure 2.SWE during active stretch of the gastrocnemius muscle in a healthy participant
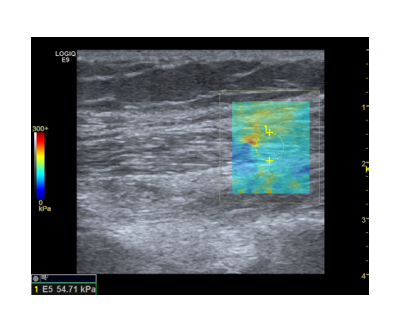
Figure 3.SWE during active stretch of the gastrocnemius muscle
in a heart failure patient (Original Image)
This heart failure patient presents an elasticity of 54.7 kPa during exercise.
The region of interest is positioned within the coloured square.
The color-coded scale on the left side measures the shear modulus in
a range from 0kPa to 300kPa.
Seventeen control patients presented a median shear modulus of 86.3 kPa compared to 59.6 kPa in the chronic heart failure group of our study [4]. The stretch exercise activated sufficiently the gastrocnemius muscle and increased blood perfusion in the corresponding muscle. The reduced muscle stiffness visualized by SWE in CHF patients correlated with diminished muscle contractility, caused by impaired local blood circulation. Other studies as the original publication of Shaap et al. propose that an increased level of inflammatory markers such as C-reactive protein or Interleukin-6 might lead to a decreased muscle mass in thigh regions of heart failure patients [26].
Last but not least, the foot dorsal extension generates a sufficient contraction in the gastrocnemius muscle and is easily performed by different patient groups despite the manifestation of the heart disease [4].
Results and Discussion
We recruited and examined twenty-eight heart failure patients in different stages of their heart failure disease. All heart failure patients exhibited significantly lower gastrocnemius elasticity than healthy participants. Using a receiver operator analysis curve (ROC-curve) we calculated a possible cut-off value of 81.1kPa for the gastrocnemius muscle during extension as a distinction between healthy persons and heart disease patients. This cut-off value was associated with a 89% sensitivity and 71% specificity [4].
Furthermore, we correlated muscle strength level with the manifestation of heart disease by dividing heart failure patients in three subgroups: NYHA-Class, NT-proBNP blood levels and left ventricle ejection fraction (LVEF). Six patients in the HFmrEF – subgroup (Heart Failure with mid-range Ejection Fraction) with a left ventricle ejection fraction (LVEF) of 40-49% performed with a median of 53 kPa followed by 61kPa in the HFrEF (Heart Failure with reduced Ejection Fraction) group with a LVEF lower than 40% [4,27]. Results changed in comparison with healthy participants with a p-value of 0.01 for HFrmrEF and p-value lower 0.001 for HFrEF (Figure 4).

Figure 4.Boxplots-Diagram “Muscle elasticity of the gastrocnemius muscle during exercise in healthy participants and patients with reduced left ventricle ejection fraction“ ( Original image) Heart failure patients exhibit significantly lower kPa-results of the lower limb muscles in both HFmrEF und HFrEF groups
Furthermore, heart failure patients were separated in two groups according to their proBNP blood level: 125-1000ng/ml and higher than 1000ng/ml. In the first group they presented a median elasticity value of 67kPa followed by 57kPa for the second subgroup. In both groups muscle elasticity was markedly reduced compared to healthy participants [4].
Heart failure patients with a slightly limited physical activity defined by NYHA-Class I and II performed with a median kPa-value of 64kPa during stretch. Patients with a severe clinical manifestation of their heart disease (NYHA Class III) demonstrated a median elasticity value of 50kPa [4,28]. Both elasticity measures were significantly reduced in comparison control group participants.
Last but not least, we observed a significant correlation between gastrocnemius muscle elasticity during contraction and heart failure characteristics such as NYHA-Class, LVEF and proBNP blood levels in a simple regression model. In another regression analysis, shear wave modulus at stretch proved to be predictive for an underlying heart failure disease with an Odds-Ratio of 0.859 (p< 0.05) [4].
Conclusion
Shear wave elastography is a novel ultrasound method, which has gained popularity in the examination of muscle tissue`s characteristics in the last few years. Our original study of 2018 presented the potential of shear wave elastography in diagnosing and even quantifying muscle weakness in chronic heart failure patients. A future study plans to evaluate the potential use of SWE in detecting muscle malperfusion and possible ischemia in intensive care patients with extracorporeal life support. Peripheral ischemia presents one of the most common complications in patients with venous-arterial extracorporeal membrane oxygenation (VA-ECMO) with incidence rates from 10% up to 70% [29-31]. We hypothesize that patients with VA-ECMO suffer from different forms of limb ischemia despite antegrade perfusion at the cannulation side. By performing passive stretching of the gastrocnemius muscle we could use shear wave elastography in detecting differences in muscle elasticity as potential signs for malperfusion and peripheral ischemia. In peripheral arterial disease (PAD) with heavily calcified blocked arteries incompressible with blood pressure cuffs, ankle-brachial (ABI) indexes cannot be assessed and thus the diagnosis of PAD cannot accurately confirmed. In this clinical situation, which frequently occurs in diabetics or patients with nephritic calcinosis, ABI and other non-invasive tools for diagnosis of PAD are unreliable. Muscle SWE might help to establish the diagnosis of PAD by demonstrating altered peripheral muscle perfusion in combination with a standard color-coded imaging of reduced blood flow velocity. Clinical studies for the diagnostic value of shear wave elastography in PAD patients are planned.
Future Perspectives
Clinical studies in patients with peripheral arterial disease (PAD) or other means of in arterial inflow obstructions of peripheral muscles using the described SWE technique are underway. It is yet unclear to what extent stable PAD contributes to a reduced peripheral muscle perfusion, especially in chronic heart failure patients.
Conflict of interest
None declared.
References
1. Yamakoshi Y, Sato J, Sato T. Ultrasonic imaging of internal vibration of soft tissue under forced vibration. IEEE Trans. Ultrason, Ferroelect, Freq Contr. 1990; 37: 45–53.
2. Yoshida K, Itoigawa Y, Maruyama Y, et al. Application of shear wave elastography for the gastrocnemius medial head to tennis leg: Application of Shear Wave Elastography. Clin Anat. 2017; 30: 114–119.
3. Nakamura M, Ikezoe T, Umegaki H, et al. Shear elastic modulus is a reproducible index reflecting the passive mechanical properties of medial gastrocnemius muscle belly. Acta Radiologica Open. 2016; 5: 1–6.
4.Maslarska, M, Weis, C, Bode, C, Hehrlein, C,2018. Shear Wave Elastography of Peripheral Muscle Weakness in Patients with Chronic Congestive Heart Failure. Ultrasound in Med Biol. 44: 2531–2539.
5. Kot, B.C.W, Zhang, Z.J, Lee, A.W.C, Leung, V.Y.F, Fu, S.N, 2012. Elastic Modulus of Muscle and Tendon with Shear Wave Ultrasound Elastography: Variations with Different Technical Settings. PLoS ONE. 7:443–448.
6. Conti, C.B, Cavalcoli, F, Fraquelli, M, Conte, D, Massironi, S, 2016. Ultrasound elastographic techniques in focal liver lesions. WJG. 22: 2647–2656.
7. Ferraioli, G, 2014. Point shear wave elastography method for assessing liver stiffness. WJG. 20: 4787.
8. EASL-ALEH Clinical Practice Guidelines: Non-invasive tests for evaluation of liver disease severity and prognosis, 2015. Journal of Hepatology 63: 237–264.
9. Hari, S, Paul, S.B, Vidyasagar, R, Dhamija, E, Adarsh, A.D, Thulkar, S, Mathur, S, Sreenivas, V, Sharma, S, Srivastava, A, Seenu, V, Prashad, R, 2018. Breast mass characterization using shear wave elastography nd ultrasound. Diagnostic and Interventional Imaging 99: 699–707.
10. Cebi Olgun, D, Korkmazer, B, Kilic, F, Dikici, A.S, Velidedeoglu, M, Aydogan, F, Kantarci, F, Yilmaz, M.H, 2014. Use of shear wave elastography to differentiate benign and malignant breast lesions. Diagn Interv Radiol 20: 239–244.
11. Hefeda, M.M, 2019. Value of the New Elastography Technique using Acoustic Radiation Force Impulse in Differentiation between Hashimoto’s Thyroiditis and Graves’ Disease. JCIS. 9: 17.
12.Botar-Jid, C, Damian, L, Dudea, S.M, Vasilescu, D, Rednic, S, Badea, R, 2010. The contribution of ultrasonography and sonoelastography in assessment of myositis. Medical Ultrasonography. 12: 120–126.
13. Leong HT, Ng GY, Leung VY, et al. Quantitative Estimation of Muscle Shear Elastic Modulus of the Upper Trapezius with Supersonic Shear Imaging during Arm Positioning. PLoS ONE. 2013; 8: e67199.
14. Nordez A, Hug F. Muscle shear elastic modulus measured using supersonic shear imaging is highly related to muscle activity level. J Applied Physiol. 2010; 108: 1389–1394.
15. Dubois G, Kheireddine W, Vergari C, et al. Reliable Protocol for Shear Wave Elastography of Lower Limb Muscles at Rest and During Passive Stretching. Ultrasound Med Biol. 2015;41, 2284–229.
16. Kot BCW, Zhang ZJ, Lee AWC, et al. Elastic Modulus of Muscle and Tendon with Shear Wave Ultrasound Elastography: Variations with Different Technical Settings. PLoS ONE. 2012; 7:443–448.
17.Gennisson JL, Catheline S, Chaffaı̈ S, et al. Transient elastography in anisotropic medium: Application to the measurement of slow and fast shear wave speeds in muscles. The Journal of the Acoustical Society of America. 2003; 114: 536–541.
18. Gennisson JL, Deffieux T, Macé, E, et al. Viscoelastic and Anisotropic Mechanical Properties of in vivo Muscle Tissue Assessed by Supersonic Shear Imaging. Ultrasound Med Biol. 2010; 36: 789–801.
19. Bortolotto C, Lungarotti L, Fiorina I, et al. Influence of subjects’ characteristics and technical variables on muscle stiffness measured by shear wave elastosonography. J Ultrasound. 2017; 20: 139–146.
20. Armstrong RB, Delp MD, Goljan EF, et al. Distribution of blood flow in muscles of miniature swine during exercise. Journal of Applied Physiol. 1987; 62:1285–1298.
21. Rowell, Loring B. Human cardiovascular control. Oxfort University Press, New York, 1993.
22. Delp MD, Laughlin MH. Regulation of skeletal muscle perfusion during exercise: Control of muscle blood flow. Acta Physiologica Scandinavica. 1998; 162: 411–419.
23. Meininger GA, Davis MJ. Cellular mechanisms involved in the vascular myogenic response. Am J Physiol -Heart Circulatory Physiol. 1992; 263: H647–H659.
24. Laughlin MH. Skeletal muscle blood Laughlin MH, Armstrong, R.B, 1985. Muscle blood flow during locomotory exercise. Exerc Sport Sci Rev 13, 95–136.
25. flow capacity: role of muscle pump in exercise hyperemia. Am J Physiol -Heart Circulatory Physiol.1987; 253:H993–H1004.
26. Schaap LA, Pluijm SMF, Deeg DJH, et al. Higher Inflammatory Marker Levels in Older Persons: Associations with 5-Year Change in Muscle Mass and Muscle Strength. The Journals of Gerontology Series A: Biological Sciences and Medical Sci. 2009; 64A: 1183–1189.
27. Ponikowski P, Voors AA, Anker SD, et al. ESC Guidelines for the diagnosis and treatment of acute and chronic heart failure: The Task Force for the diagnosis and treatment of acute and chronic heart failure of the European Society of Cardiology (ESC)Developed with the special contribution of the Heart Failure Association (HFA) of the ESC. Eur Heart J. 2016; 37: 2129–2200.
28. Dolgin, M, New York Heart Association (Eds). Nomenclature and criteria for diagnosis of diseases of the heart and great vessels, 9. ed, 3. print. ed, A Little, Brown handbook. Little, Brown and Co, Boston, Mass. 1994; ISBN 978-0-316-60538-0
29. Bonicolini E, Martucci G, Simons J, et al. Limb ischemia in peripheral veno-arterial extracorporeal membrane oxygenation: a narrative review of incidence, prevention, monitoring, and treatment. Crit Care. 2019; 23: 266.
30. Yen CC, Kao CH, Tsai CS, et al. Identifying the Risk Factor and Prevention of Limb Ischemia in Extracorporeal Membrane Oxygenation with Femoral Artery Cannulation. HSF. 2018; 21: 018.
31. Bisdas T, Beutel G, Warnecke G, et al. Vascular Complications in Patients Undergoing Femoral Cannulation for Extracorporeal Membrane Oxygenation Support. Ann Thorac Surg. 2011; 92: 626–631.
Received: September 28, 2021;
Accepted: October 04, 2021;
Published: October 06, 2021.
To cite this article : M. Maslarska, C. Bode, C. Hehrlein. Shear Wave Elastography as a Novel Ultrasound Method for Monitoring Exercise Capacity of Patients with Congestive Heart Failure. British Journal of Heart Diseases. 2021; 3:1
©2021 M Maslarska, et.al.